In the modern era of construction and engineering, sustainability has become a pivotal concern. Buildings and infrastructure projects are no
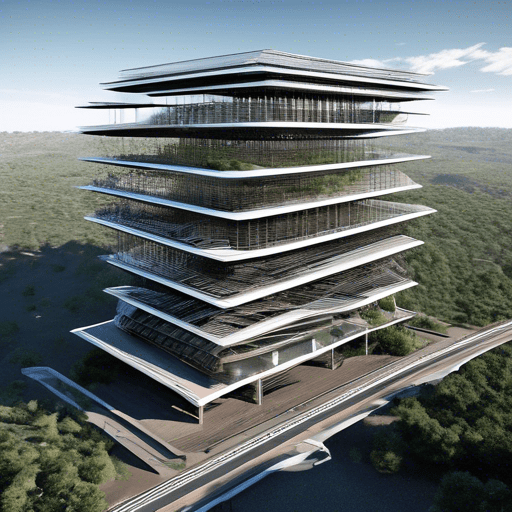
In the field of structural engineering, precision and safety are not just desirable attributes – they are fundamental requirements. The structures we design and build, whether they are towering skyscrapers, sprawling bridges, or intricate industrial facilities, play a crucial role in the safety and well-being of the people who inhabit or use them. A single structural failure can have catastrophic consequences, leading to loss of life, property damage, and significant economic and social disruption.In this article we will be informed about some computational techniques in structural engineering.
As such, structural engineers have a profound responsibility to ensure that their designs are not only aesthetically pleasing and functionally efficient but also meticulously engineered to withstand the rigors of the real world. This means accounting for a wide range of factors, from environmental conditions and seismic activity to the complex interplay of forces and stresses that act upon a structure over its lifetime.
Historically, structural engineers have relied on a combination of empirical data, theoretical models, and hands-on experience to inform their designs. While these traditional methods have served the industry well, the ever-increasing complexity of modern structures and the growing demand for more efficient and sustainable solutions have necessitated the development of more advanced computational techniques.
Conventional structural engineering practices have long been anchored in a combination of analytical calculations, physical testing, and expert judgment. Engineers would painstakingly work through complex equations, often relying on simplified assumptions and approximations to model the behavior of a structure. This approach, while effective in many cases, had its limitations, particularly when dealing with highly complex or unconventional designs.
The advent of advanced computational techniques has revolutionized the way structural engineers approach their work. By leveraging the power of modern computing and sophisticated algorithms, these techniques enable engineers to simulate and analyze the behavior of structures with unprecedented accuracy and detail.
At the heart of this computational revolution are tools like finite element analysis (FEA), computational fluid dynamics (CFD), and artificial intelligence (AI)-powered algorithms. These technologies allow engineers to create detailed digital models of structures, simulate their response to various loads and environmental conditions, and optimize their designs to ensure maximum safety and performance.
Finite element analysis is a powerful computational technique that has become a cornerstone of modern structural engineering. FEA allows engineers to break down complex structures into smaller, manageable elements, each with its own set of material properties, boundary conditions, and loading scenarios. By analyzing the behavior of these individual elements and how they interact with one another, FEA can provide a comprehensive understanding of the overall structural response.
One of the key advantages of FEA is its ability to handle highly complex geometries and loading conditions. Traditional analytical methods often rely on simplifying assumptions that may not capture the true behavior of a structure, particularly in cases where there are irregular shapes, complex load distributions, or nonlinear material properties. FEA, on the other hand, can account for these complexities, enabling engineers to make more accurate predictions and optimize their designs accordingly.
Moreover, FEA allows for the simulation of a wide range of structural phenomena, from static and dynamic loads to thermal effects and material fatigue. This flexibility enables engineers to explore various “what-if” scenarios, testing the limits of a structure’s performance and identifying potential weaknesses or failure modes before construction even begins.
While FEA focuses on the structural integrity of a MEP design, computational fluid dynamics (CFD) plays a crucial role in enhancing the safety and performance of structures by analyzing the behavior of fluids, such as air and water, interacting with the structure.
In the context of structural engineering, CFD can be used to simulate the effects of wind, rain, and other environmental factors on a building or infrastructure. By modeling the flow of these fluids around and through a structure, engineers can better understand the forces and pressures acting upon it, allowing them to optimize the design for improved aerodynamics, wind resistance, and water management.
CFD also has applications in the analysis of structural vibrations and the assessment of seismic performance. By simulating the dynamic behavior of a structure under various loading conditions, engineers can identify potential resonance frequencies, mitigate the risk of excessive vibrations, and ensure that the structure can withstand the forces generated by earthquakes or other natural disasters.
The integration of artificial intelligence and machine learning algorithms has further enhanced the capabilities of advanced computational techniques in structural engineering. These technologies enable engineers to leverage vast amounts of data, both from past projects and real-time monitoring systems, to identify patterns, detect anomalies, and make more accurate predictions.
For example, AI-powered algorithms can be trained on historical structural performance data to develop predictive models that can anticipate the behavior of a structure under different loading scenarios. This allows engineers to proactively identify potential weaknesses or failure points, leading to more robust and resilient designs.
Machine learning algorithms can also be used to optimize the design process itself, analyzing the performance of various design iterations and suggesting improvements to enhance efficiency, safety, and cost-effectiveness. By automating certain aspects of the design workflow, these AI-driven tools can help engineers save time and resources while maintaining the highest standards of quality and precision.
Moreover, the integration of AI and ML with real-time monitoring systems can enable the continuous assessment of a structure’s condition, allowing for the early detection of issues and the implementation of preventive maintenance strategies. This can significantly extend the lifespan of a structure and reduce the risk of catastrophic failures.
The implementation of advanced computational techniques in structural engineering has yielded numerous benefits, but it has also presented some challenges that engineers must navigate.
One of the primary advantages of these techniques is the enhanced precision and accuracy they bring to the design process. By simulating the behavior of structures with greater fidelity, engineers can identify potential weaknesses, optimize designs, and ensure that their projects meet or exceed safety standards. This not only improves the overall quality of the final product but also reduces the risk of costly failures or delays during construction.
Another key benefit is the ability to explore a wider range of design alternatives and scenarios. With traditional methods, engineers were often limited by the time and resources required to physically test or analytically model each iteration. Advanced computational techniques, however, allow for the rapid evaluation of multiple design options, enabling engineers to identify the most optimal solution more efficiently.
Furthermore, the integration of AI and ML algorithms has introduced a new level of intelligence and automation to the design process. By learning from vast datasets and identifying patterns in structural behavior, these technologies can provide valuable insights that would be challenging for even the most experienced engineers to uncover on their own. This can lead to more innovative and cost-effective solutions, as well as the ability to anticipate and mitigate potential issues before they arise.
However, the implementation of advanced computational techniques is not without its challenges. One of the primary hurdles is the need for specialized expertise and training. Effectively utilizing these tools requires a deep understanding of the underlying algorithms, computational models, and software platforms, which can be a significant investment for many engineering firms.
Another challenge is the sheer volume of data and computational power required to run complex simulations and analyses. This can place a significant strain on an organization’s IT infrastructure and may necessitate the adoption of cloud-based computing or high-performance computing resources.
Additionally, there is the question of validation and trust in the results generated by these advanced computational techniques. While they offer unprecedented levels of precision and detail, engineers must be diligent in verifying the accuracy of their models and ensuring that the underlying assumptions and inputs are valid. Failure to do so can lead to flawed decision-making and potentially disastrous consequences.
As the field of structural engineering continues to evolve, the importance of advanced computational techniques in enhancing precision and safety will only grow more pronounced. These powerful tools have already transformed the way engineers approach the design, analysis, and optimization of complex structures, and their potential impact on the industry is poised to expand exponentially in the years to come.
From the seamless integration of FEA, CFD, and AI-driven algorithms to the development of real-time monitoring and predictive maintenance systems, the future of structural engineering is undoubtedly computational. By harnessing the power of these advanced techniques, engineers will be able to push the boundaries of what is possible, creating structures that are not only safer and more resilient but also more efficient, sustainable, and cost-effective.
As the industry continues to grapple with the challenges of an increasingly complex built environment, the need for innovative solutions that prioritize precision and safety will only become more pressing. Advanced computational techniques will play a crucial role in meeting this demand, empowering structural engineers to design and build the infrastructure that will shape the world of tomorrow.
In conclusion, the integration of advanced computational techniques in structural engineering represents a transformative shift in the way we approach the design and construction of our built environment. By leveraging the power of these powerful tools, engineers can enhance the precision and safety of their projects, ultimately contributing to the creation of a more resilient and sustainable future for all.
About Author
Xhuljo Jakup